 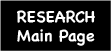

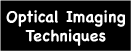
 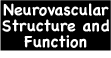
 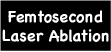

|
|
Neurovascular Structure and Function
Background : Cerebral Blood Flow and Angioarchitechture
Blood is a limited
but vital resource for the brain. Normal neuronal
activity and proper brain physiology are dependent on
the neurovascular blood supply which provide the neurons
and glia with oxygen, glucose, and waste removal. In
addition to understanding its role in normal brain
homeostasis, the study of cortical vasculature is
important with regards to many neurological diseases
with associated vascular pathology. In particular,
stroke is currently the 4th leading cause of death in
America, responsible for 130,000 deaths per year.
Finally, a growing number of techniques utilize blood
oxygenation measurements as a surrogate for measuring
neuronal activity. This includes widely-used techniques
such as intrinsic optical imaging (IOS) and
blood-oxygenation level dependent functional magnetic
resonance imaging (BOLD fMRI).
Yet despite the
importance of the neuronal vasculature in both research
and clinical contexts, the microvascular details of
cortical blood flow have, until recently, remained
largely unquantified. Notably, no large-scale
quantitative maps of the complete neurovascular
connectivity exist to provide insights into the dynamics
of blood flow and blood perfusion within the brain.
Over the past
decade, my colleagues and I have endeavored to elucidate
some of the microscopic details of blood flow in the
brain by undertaking research in two complementary
directions: 1) In vivo imaging and perturbation
of blood flow with micrometer resolution and accuracy,
and 2) 3-D post-mortem quantitative
reconstruction of cortical angioarchitecture to compute
and predict patterns of blood flow across
millimeter-scale regions of mouse cortex.
|
3-D Histology of Angioarchitecture
Futher intepretation of the flow data obtained from our in
vivo imaging studies as well data
from other methods that utilize blood flow and blood
oxygenation requires a detailed map of cerebral vascular
connectivity. To that end, we have used our
All-Optical Histology technique to perform in-situ
block-face imaging of vascular-labeled cortical
tissue. From these data, we are able to reconstruct
the full connectivity of the surface and sub-surface
vascular network across a volume that is 2 - 3 millimeters
on edge, and spans the depth of cortex.
Simultaneously, we label and record the position of every
neuronal and non-neuronal somata within the tissue
volume. This allows us to correlate the vascular
network to the surrounding neuronal tissue and any
organizational units within that tissue, such a the vibrissa
barrels in primary somatosensory cortex.
Using code developed specifically for this
purpose, we are able to extract a fully-connected vectorized
map of the cerebral vascular network within these
cubic-millimeter-scale tissue volumes. By carefully
measuring the radius of each vascular segment within the
network, and applying a previously-determined relationship
between vessel diameter and fluid resistance to blood of a
known hematocrit, we are able to produce deterministic
resistive model of the flow across the reconstructed
network. In silica experiments in which the
change in flow is measured after manipulation to a single
vessel resistance yield flow patterns that closely match
those found in previous in vivo experiments of the
same nature.
From these reconstructions, we are able to conclude that,
within the vibrissa barrel cortex, the microvascular
angioarchitechture represents a fully-connected continuous
network. This stands in contrast to the previously
held-notion of (semi-) discrete neurovascular units.
We find no evidence of such discrete units within the
vascular architecture. Although we do find that flow
sourced from a single penetrating arteriole is constrained
to a finite lateral extent within the cortex, the "confined
lateral flow" show no correlation to underlying cortical
columns or "barrels". Furthermore, we are able to
present a simple model of blood flow in the cortex that
accounts for this lateral confinement of flow within the
fully interconnected network. We find that the fluid
resistance of the venules to be smaller than the effective
net resistance of the microvascular network. Hence,
the distribution of high pressure sources (penetrating
arterioles) and low resistance sinks (arising venules)
result in watershed domains that limit the extent of flow
from any single source.
|